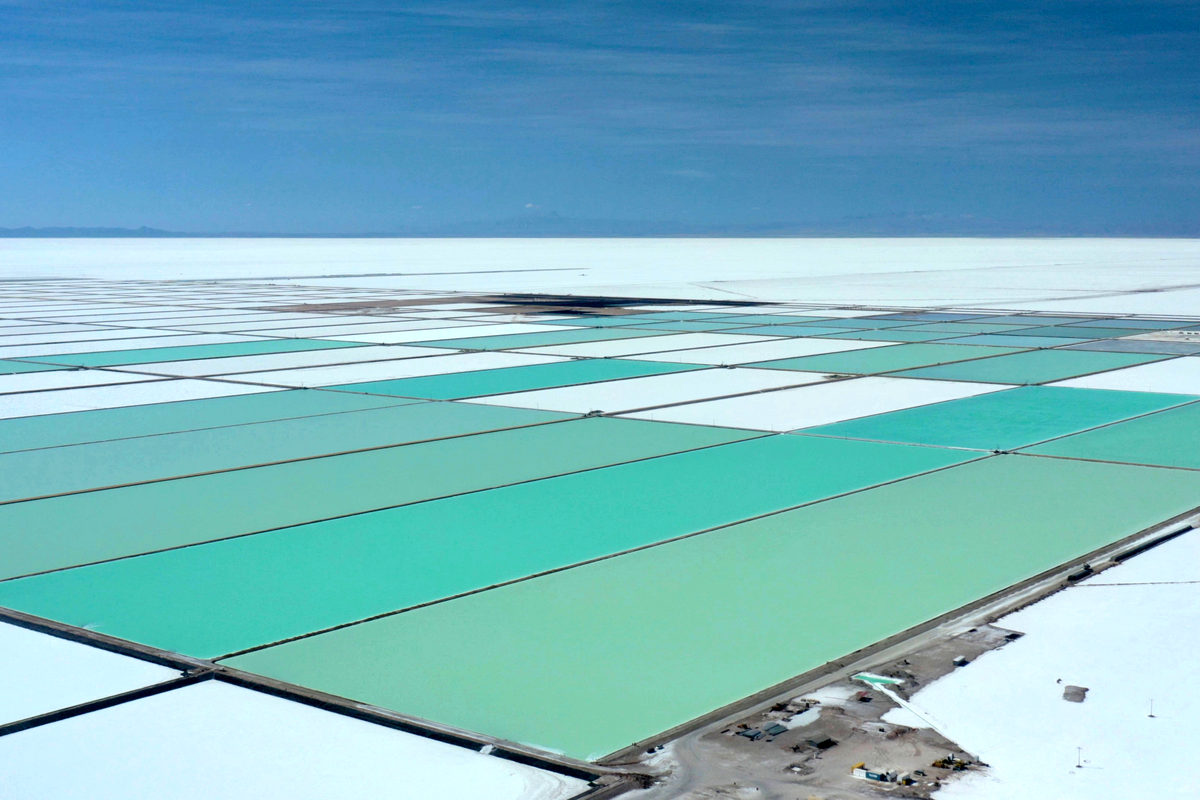
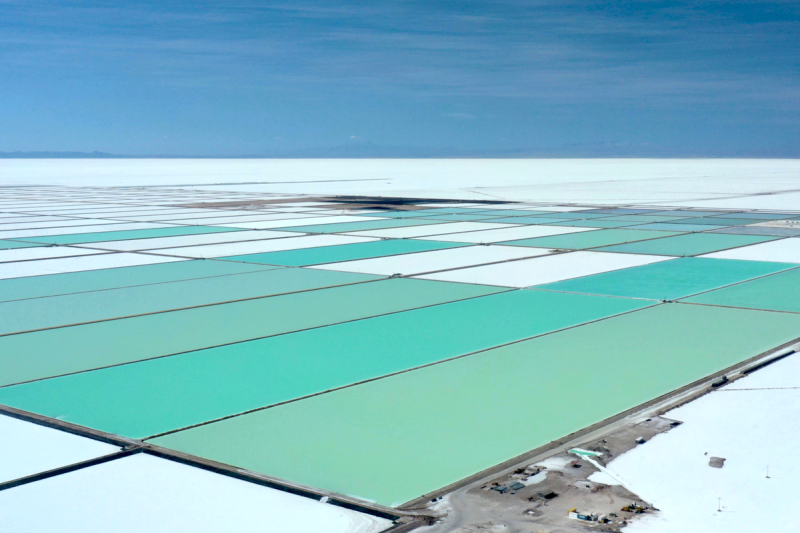
Many years ago, I read an article about the new hotness: lithium batteries. The author opened with what he no doubt thought was a clever pop culture reference by saying that the mere mention of lithium would "strike fear in the hearts of Klingons." It was a weak reference to the fictional "dilithium crystals" of Star Trek fame, and even then I found it a bit cheesy, but I guess he had to lead with something.
Decades later, while a deeper understanding of the lore makes it clear that a Klingon's only fear is death with dishonor, there is a species here on earth that lives in dread of lithium: CEOs of electric vehicle manufacturing concerns. For them, it's not the presence of lithium that strikes fear, but the relative absence of it; while it's the 25th most abundant element in the Earth's crust, and gigatons are dissolved into the oceans of the world, lithium is very reactive and thus tends to be diffuse, making it difficult to obtain concentrated in the quantities their businesses depend on.
As the electric vehicle and renewable energy markets continue to grow, the need for lithium to manufacture batteries will grow with it, potentially to the point where demand outstrips the mining industry's production capability. To understand how that imbalance may be possible, we'll take a look at how lithium is currently mined, as well as examine some new mining techniques that may help fill the coming lithium gap.
A Rocky Start
Although lithium has been known and well-characterized by chemists since the early 1800s, it was only in the middle of the previous century that commercial uses for lithium compounds were identified. The aircraft industry's demand for stable lubricants resulted in the development of greases made from lithium soaps, and the need for high-performance but lightweight metals led the aluminum industry to employ lithium to improve the Hall-Héroult smelting process. Around the same time, doctors discovered that lithium salts can treat patients with bipolar disorder.
A large crystal of spodumene (lithium aluminum inosilicate, LiAl(SiO3)2) found in Massachusetts. Source: by Rob Lavinsky, iRocks.com – CC-BY-SA-3.0
Even with the additional demand of the nascent nuclear industry starting in the 1940s, pretty much all the lithium needed could be supplied from small hard-rock mining operations that exploited deposits of rocks containing large crystals of lithium minerals, like spodumene, petalite, and lepidolite. These three minerals remain in high demand to this day for the production of lithium hydroxide, one of the two main lithium compounds used by industry.
The production of lithium from hard rock mines has a lot in common with other mining and refining methods we've discussed in this series. Ore-bearing rocks are blasted out of open-pit mines, scooped up by gigantic loaders, and trucked to a refining plant. There, the rock is reduced in size by a series of crushers and mills until it becomes a fine powder. Water is added to the powder to create a slurry known as pulp, which also contains surfactants and dispersants that make the lithium-containing minerals hydrophobic. In a shallow tank with air pumped through from the bottom, the light lithium forms a froth that floats to the top while the heavier rock particles sink.
After the lithium froth is skimmed off the flotation tank, the extra liquid is filtered off to create a concentrated but impure lithium powder that needs to be refined. The refining process depends a lot on the source minerals and desired end product, but for concentrated spodumene ore, lithium is typically leached out using a combination of sulfuric acid and sodium hydroxide. While this is a direct route with high yields, the acids and bases involved can make it environmentally problematic. Other acid-free leaching processes have been developed as a result, which is said to be the kind of process Tesla is using in their new lithium hydroxide plant being built next to their Texas Gigafactory.
Down in the Brine Mine
As mentioned before, seawater contains something like 230 billion tonnes of lithium, dissolved mainly as lithium salts. While this constitutes the bulk of the lithium on the planet, it's far too diffuse -- a mere 25 micromolar -- to serve as a viable commercial source without vast expenditures of energy to extract and concentrate it. But seawater isn't the only brine that contains lithium, and extracting the valuable metal from underground brines has become the main production method since the 1990s.
By far the biggest lithium-bearing brines are found in the "Lithium Triangle" of South America. Occupying parts of Chile, Bolivia, and Argentina, the area is home to large salt flats or salars , areas where ancient lakes or ponds evaporated, leaving behind salts and other precipitated minerals. These salt flats have built up over millions of years, leaving rich layers of minerals beneath their surfaces. And as we'll see, the flat terrain and harsh arid conditions on the surface also play a part in the mining process.
Brine ponds at the Salar de Atacama in Chile, as seen from space. For scale, each of the long, skinny ponds in the center is nearly a kilometer long. Source: NASA Earth Observatory, by Lauren Dauphin
Mining lithium brine is quite unlike any of the other methods of mining we've covered before, and couldn't be simpler. Instead of digging up rocks and painstakingly isolating the material of interest, brine mining consists of injecting water down into salt deposits through deep boreholes. The water dissolves the salt deposits, creating a rich brine that can be pumped up to the surface. The brine is pumped into shallow ponds and is left in the sun to evaporate.
When most of the water in a pond has evaporated -- up to two years later -- the now concentrated brine is harvested. The concentrate contains a variety of elements in addition to lithium, including sodium, magnesium, phosphates, and boron. The concentrate can either be further processed on-site, or as is becoming increasingly common, shipped via pipelines to ports for transport to lithium processing plants abroad.
On the face of it, the evaporation method for lithium brine mining seems like a winner. It's super simple, it's powered almost exclusively by the sun, and it's devoid of some of the impacts that a large open-pit mining operation can have. But there are still huge problems with evaporation concentration. First off, it requires vast amounts of water to create the brines in the first place, and because evaporation ponds are only practical in places where it doesn't rain much, water is already in short supply. The water used for brine mining is also lost to the atmosphere, coming back to the surface somewhere far from the evaporation ponds. Plus, the evaporation ponds occupy unbelievably large amounts of land -- some pond complexes cover an area the size of Manhattan -- which makes it difficult to scale up operations. And the amount of time it takes the sun to do its work is a problem in terms of production flexibility.
A Better Way
To make the most of brine mining while mitigating its shortcomings, direct lithium extraction methods are becoming increasingly popular. In DLE, brine is pumped from underground sources, but instead of concentrating the brine by open evaporation, lithium is removed from the brine using a number of chemical and physical methods. One method is ion-exchange adsorption, where the brine is mixed with an absorbent material that preferentially binds lithium compounds over the other compounds in the brine. One class of sorbents used in DLE is known as layered double hydroxides (LDH), materials with a layered structure that allows lithium chloride in the brine to fit between the layers while excluding the potassium, magnesium, and other salts. The brine is returned to the ground, while the high-purity lithium chloride is washed off the sorbent.
Other DLE methods include membrane-separation technologies like reverse osmosis, where the brine is pumped at high pressure through membranes with pores that retain the lithium salts, or by solvent extraction, where organic solvents are used to extract the lithium. The common theme with DLE methods, though, is the fact that they are closed-loop processes -- the water used to create the brine is returned to the underground formations containing the lithium. DLE plants also take up a fraction of the physical space that even a single evaporation pond would take, and they don't rely on extreme environments like salars to work.
Best of Both Worlds
As attractive as DLE technology is, at the scale needed to be commercially viable, DLE plants still require a fair amount of energy to run. But in some places, a quirk of geology has left ample lithium deposits near a source of abundant renewable energy. In the Imperial Valley of California lies the Salton Sea, an inland saline lake that lies atop a series of active geological faults, including the famous San Andreas fault. The area is perfect for geothermal electricity production, with eleven plants currently producing 2,250 MW. Some of these geothermal plants are co-located with DLE plants, which pump up hot, lithium-rich brines that are purified using the geothermal energy produced on-site. Environmentally speaking, such plants are about as low-impact as lithium production can be, with the geothermal DLE plant being built by Australian company Controlled Thermal Resources predicted to produce 68,000 tonnes of battery-grade lithium by 2027.
With the demand for lithium set to soar, the ability to extract what we can from the limited sources we have available using the lowest amount of energy possible is becoming a challenge indeed. Geothermal DLE seems like a good start, but the number of places in the world with both the correct geochemistry and the tectonics to support such operations is limited. It's going to take some clever engineering to get at the rest of the lithium that's available, at least with the technology and energy resources we currently have.
[Banner photo by PABLO COZZAGLIO/AFP via Getty Images]
#chemistryhacks #greenhacks #hackadaycolumns #battery #extraction #lithium #mining #renewableenergy